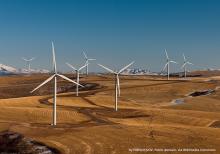
1. What is energy
The word energy derives from the Ancient Greek and was probably first introduced by Aristotle in the 4th century BC. The term identified a qualitative philosophical concept, to indicate a state of mind rather than a physical quantity.
Vincenzo Balzani, an Italian chemist who is hemeritus professor at the University of Bologna, once stated:
If you want to abash someone ask him: “what is energy”? Everybody thinks of knowing it, however, nobody will give you a clear and unique answer. We know that energy is mandatory for every process, and that energy appear in different forms (chemical, electro-magnetical, electrical, thermal, mechanical, nuclear). Reversible conversion process exists between the form (but not to nuclear energy). Energy conversion is the only way to “produce” energy, and during conversion the “quantity” is saved, while the “quality” degrades. Energy is causing wars and is the real “power” of the world. We know that is such to produce changes (material or immaterial). We know everything of “energy”, but we cannot define it. It is a quantity of universal nature that appears in material and immaterial forms, and that cannot be “reduced” to a more elemental form.
Today, energy is defined in physics as the quantitative property that must be transferred to a body or physical system to perform work on the body, or to heat it. Simply put, energy is the ability to do work. It is measured in joule, which is the energy transferred to an object by the work of moving it a distance of one meter against a force of one newton.
Energy, water and food are considered primary resources for ensuring human well-being. In particular, energy is essential to life. The sun, directly or indirectly, is the source of all the energy available on Earth and used by people, animals, plants, and microorganisms. This energy may come directly, such as in the form of radiation and photosynthesis, or indirectly, for example in the form of fossil fuels, which long ago trapped the energy of the sun that is released when they are burned. The management of energy by humans impact Earth's systems in ways that may be not completely understood, therefore energy production is to be planned carefully.
To emphasise the importance of energy, we may provide examples of energy consumption for producing essential goods for the human life:
- The production of 1 kilogram of meat on average requires 7 liters of oil; We may desalinate 1000 liters of seawater with 3 liters of oil; Producing a ton of aluminum requires about 5 tons of oil; Producing a car requires about 3 tons of oil, that is, about 25% of energy consumed by a car in its lifetime; Energy production accounts for about 75% of global greenhhttps://ourworldindata.org/ghg-emissions-by-sectorouse gas emissions, that is mainly obtained by burning fossil fuels, with a clear impact on climate change and public heath.
2. Energy transition
The recent history has shown once again the essential role of energy for international cooperation and climate change mitigation. The political crisis in Europe forced several countries to increase energy production through the use of fossil fuels and, at the same time, accelerate the energy transition.
Energy transition is a transformation of an energy system which implies a structural change in both energy supply and consumption. It implies a broad shift in technologies for energy production. Today, we use the term to indicate a transition to sustainable energy in order to mitigate climate change through the reduction of the use of fossil fuels. A previous energy transition took place during the industrial revolution and involved a larger use of coal - and later oil and natural gas - for energy production.
A relevant question about energy transition is timing (see Figure 1). In fact, the implementation of the above structural changes, which need to be supported by scientific advance and innovative technology, requires time, which may be incompatible with the targeted reductions of human emissions.
Figure 1. Possible timeline for energy transition. From Wikimedia Commons. Click here for higher resolution.
Energy transitions is a complex process as energy systems are today interconnected at the international level. Therefore, changing them requires a significant effort in term of diplomacy and global cooperation. Historical changes of energy systems have been extensively studied but conditions at actual time are much different with respect to the past. To better understand the complexity of energy transition we need to learn more on how energy is produced and used.
3. Forms of energy and conversion
There are six forms of energy (Figure 1): thermal, chemical, electrical, electromagnetic (light), kinetic (mechanical), nuclear. Chemical energy is the one that is stored by fossil fuels. Electrical can be considered a subsystem of electromagnetic. Antrophogenic and natural conversion processes allow to transform energy from one form to another.
Figure 1. Forms of energy and conversion. Natural processes are indicated in red. Black processes are artificial.
We as human are capable of performing all the transformation processes indicated in Figure 1. Energy transformation is regulated by the energy conservation law, that is, the first principle of thermodynamic: the total energy of a isolated systems remains constant. It can only be converted among the six forms. Therefore, the expression "energy production" is formally wrong. Any process is a energy conversion.
However, conversion implies a degradation of energy. Namely, energy is conserved after transformation but its capacity to produce work decreases. In fact, the second law of thermodynamics deals with energy transformations and the natural tendency to move towards more degraded forms, which cannot be used any longer. This does not mean that the total quantity of energy present in the universe is decreasing, rather that its ability to work is. The thermodynamic function assessing the degree of energy dispersion is called “entropy” . Entropy in the universe tends to increase to reach a state of balance in which the total degradation of energy is achieved corresponding to the complete inability to work. Fortunately, biological systems are open systems, which thanks to the incoming of energy from outside, restore the positive global energetic balance.
A second interesting classification of energy divides it in two categories: primary energy and secondary energy. Primary energy consists of unconverted or original fuels. Secondary energy includes resources that have been converted or stored. For example, primary energy sources include petroleum, natural gas, coal, biomass, flowing water, wind, and solar radiation. Those are the fuels that can be mined, reaped, extracted, harvested, or harnessed directly. Secondary energy cannot be harnessed directly from nature; rather, secondary energy is energy that has already been converted. For example, electricity cannot be mined or harvested, though it is available in quick bursts on occasion from lightning. It is generated as a secondary form from primary fuels, like natural gas. Primary energy can be non-renewable or renewable. Renewable energy is collected from renewable resources that are naturally replenished on a human timescale. It includes sources such as sunlight, wind, rain, tides, waves, and geothermal heat. Renewable energy stands in contrast to fossil fuels, which are being used far more quickly than they are being replenished. Although most renewable energy sources are sustainable, some are not. For example, some biomass sources are considered unsustainable at current rates of exploitation.
Primary energy can be broadly classified into three parts (see Figure 2):
- Crude oil, hard coal, natural gases, Nuclear energy etc;
- Waste;
- Wind, geothermal, biomass, hydroelectricity, wave and tidal energy etc..
Note that hydroelectricity is meant to indicate energy contained in flowing water (water power) and therefore it is primary. Primary energy is measured in TOE (tons of oil equivalent).
Figure 2. Primary and secondary energy. Source: Watt Watchers of Texas.
3. Global energy budget and greenhouse gases emissions
Energy production is the driver of most of the global emissions of greenhouse gases. In fact, recent assessments indicate the following estimates (see also Figure 3):
- Energy (electricity, heat and transport): 73.2%;
- Direct Industrial Processes: 5.2%;
- Waste: 3.2%;
- Agriculture, Forestry and Land Use: 18.4%.
Figure 3. Greenhouse gases emissions by sector.
Most of the energy is still produced by burning fossil fuels. Renewables are increasing but they still remain a limited energy source (Figure 4 and 5).
Figure 4. World primary energy use by fuel type.
Figure 5. World energy balance.
Half of global energy is produced by China, the United States and the Arab states of the Persian Gulf. The Gulf States and Russia export most of their production, largely to the European Union and China where not enough energy is produced to satisfy demand. Energy production increases slowly, except for solar and wind energy which grows more than 20% per year. Renewable energy is emerging as a valuable opportunity to decrease global emissions for energy production, but in turn it entails a significant environmental impact. For instance, wind farms are responsible for noise and visual pollution; solar farms occupy a significant fraction of land and hydropower has an impact on the water cycle and land occupation, besides implying social risks.
Energy is processed to make it suitable for consumption by end users. The supply chain between production and final consumption involves many conversions and much trade and transport among countries, causing a loss of one quarter of energy before it is consumed. It is interesting to note that the global final energy use is given by the figures that follow (source: International Energy Agency, 2017):
- Residential: 21%;
- Transport: 29%;
- Industry: 29%;
- Other: 21%.
Figure 6 shows a picture of the progress from 2000 to 2020 of global energy use by fuel type.
Figure 6. Progress from 2000 to 2021 of global energy use by fuel type.
Figure 7 shows the total energy consumption by source in 2012.
Figure 7. Total world energy consumption by source in 2012. By Delphi234 - Own work, CC0, https://commons.wikimedia.org/w/index.php?curid=20033816
Energy consumption per person in North America is very high while in developing countries it is low and more renewable. There was a significant decline in energy usage worldwide caused by the COVID-19 pandemic, notably in the iron and steel industry as demand for new construction shrank. To reach levels similar to that in 2019, there would need to be an increase in the global demand for manufactured goods by the iron and steel industry (for more information see the page "World energy supply and consumption" in Wikipedia).
Furthermore, it is significant to note that energy production and consumption is enormously differentiated with respect to several regions of the world therefore highlighting a significant disparity.
4. Renewable energy
Renewable energy will play a key role in the decarbonisation of our energy systems in the coming decades. Renewable energy is collected from resources that are naturally replenished on a human timescale, and therefore does not include fossil fuels, which are being used far more quickly than they are being replenished. It includes sources such as solar energy, wind, rain, tides, waves, and geothermal heat. Although most renewable energy sources are sustainable, some are not. For example, some biomass sources are considered unsustainable at current rates of exploitation.
About 20% of humans' global energy consumption is covered by renewable energy, including almost 30% of electricity. Most countries are expanding their sources of renewable energy (see Figure 8).
Figure 8. Renewable energy capacity additions in 2020 expanded by more than 45% from 2019, including 90% more new wind power (green) and a 23% expansion of new solar photovoltaic installations (yellow). From https://en.wikipedia.org/wiki/Renewable_energy. By RCraig09 - Own work, CC BY-SA 4.0, https://commons.wikimedia.org/w/index.php?curid=105192777
Figure 9 shows the total energy production by countries, highlighting a still heterogeneous distribution.
Figure 9. Total energy production by countries. The left vertical axis refers to the whole world, while the right vertical axis refers to the individual countries. Yellow is China; brown is Russia; light blue is Africa; blue is United States; green is Europe; dark green is Central and South America. By Delphi234 - U.S. Energy Information Administration (EIA)website eia.gov, Public Domain, https://commons.wikimedia.org/w/index.php?curid=36175030
Most of renewable electric energy is generate by hydropower (see Figure 10).
Figure 10. Renewable energy generation by source. From https://en.wikipedia.org/wiki/Renewable_energy.
Hydropower is an essential resource for its flexibility, as generation can be controlled in time and therefore is an ideal integration of other energy sources that are less flexible. Furthermore, hydropower can be easily stored and pumped back in the reservoir, therefore giving further opportunities for enhancing flexibility. However, hydropower is generating concerns related to its sustainability, as reservoirs are subjected to siltation with limited options for recovery. Furthermore, hydropower is generating a significant environmental impact, including effects on groundwater resources and local and global climate. Finally, hydropower is entailing risks for the communities exposed to floods induced by dam break and mismanagement of the storage capacity.
Figure 11. The Three Gorges Dam on the Yangtze River in China.
Renewable energy potential is characterised by a heterogeneous distribution (see Figure 12 and 13).
Figure 12. Wind energy generation by region over time.
Figure 12. Global map of wind power density potential.
The same heterogeneity is observed for solar energy (Figure 14).
Figure 14. Global map of horizontal irradiation.
Heterogeneity create disparities in countries' accessibility to renewable resources.
Production of solar energy through photovoltaic systems implies a significant environmental impact, related to loss of land for agricultural production and changes in the water cycle for the reduced infiltration (see Figure 15). The cost for solar energy production, on the other hand, is steadily decreasing (Figure 16).
Figure 15. Komekurayama photovoltaic power station in Kofu, Japan. By RCraig09 - Own work, CC BY-SA 4.0, https://commons.wikimedia.org/w/index.php?curid=138292064.
Figure 16. Swanson's law–stating that solar module prices have dropped about 20% for each doubling of installed capacity—defines the "learning rate" of solar photovoltaics. By Sakaori (talk) - Own work, CC BY-SA 3.0, https://commons.wikimedia.org/w/index.php?curid=18168398.
5. Nuclear energy
Nuclear energy is the subject of a public debate since its introduction, which represented a step change to address the increasing demand for energy and electricity. Indeed, in view of the increasing population we expect that the global need for electricity will increase to a level that may not be sustainable by the current energy sources, even if future savings are properly considered. Nuclear energy, introduced in the 1950s, is a possible solution.
The start of the nuclear age was not easy from a technical point of view. It involved a substantial body of research starting from the discovery of nuclear fission in 1938 and the creation of the first man-made nuclear reactor, the Chicago Pile-1, in 1942, with an experiment led by Enrico Fermi. Later on, after nuclear energy became a reality and several nuclear plants were built, critical opinions emerged about plant safety, which were reinforced after the accidents occurred in 1979 (Three Mile Island reactor, TMI, in the United States ) and 1986 (Chernobyl reactor in the Soviet Union). These two accidents caused a slow down in the Western world of nuclear power production, while in Asian countries such as Japan, the Republic of Korea, Taiwan and, more recently, China and India the production of nuclear energy kept increasing.
Today nuclear power is getting again increasing suggestion at the global level as it is seen as a fundamental step to cope with future energy demands and climate change. Indeed, as for greenhouse gases emissions, nuclear energy is clean and can be modulated, therefore being an interesting solution to cope with the intermittency of solar and wind energy.
A nuclear reactor is a complex machine that can be built following different methods. Its fundamental component is the core, where the fuel is found which is essentially uranium-based. The nuclear reaction - through fission - produces heat that is then used to produce steam to activate turbines to produce electricity like in any thermoelectric plant. In the core there is also the moderator, to reduce the energy of the fission neutrons, and the heat transfer fluid, to transport the heat outside the core (Figure 17 and 18).
Uranium is a very efficient fuel, generating approximately ten thousand times more energy than that generated by an equal mass of fossil fuel. However, it too is consumed, and therefore nuclear energy depends on the abundance of this raw material.
5.1. Nuclear fission
Nuclear fission is a particular disintegration process during which heavy nuclei, such as those of uranium or thorium, if suitably bombarded with neutrons, split into two fragments, both positively charged, which violently repel each other and move away with high energy kinetics. With fission, neutrons are also released which can in turn induce other fissions, triggering the so-called chain reaction which, in a nuclear reactor, allows it to be kept running by producing energy continuously and constantly.
With around 440 reactors operating in 30 different countries, nuclear fission accounted for 10% of global electricity production at the end of 2019.
5.2. Nuclear fusion
Fusion is the nuclear reaction that occurs in the sun and other stars, with the production of an enormous quantity of energy: two nuclei of light elements, such as deuterium and tritium, at high temperatures and pressures, fuse to form nuclei of heavier elements such as helium with emission of large quantities of energy. The two nuclei can only fuse at very short distances, for this to happen the speed at which they collide must be very high: their kinetic energy (and therefore the temperature) must be very high.
To obtain fusion reactions in the laboratory it is necessary to bring a mixture of deuterium and tritium to very high temperatures (100 million degrees) for sufficiently long times. At very high temperatures the individual particles of a gas tend to dissociate into the constituent elements (ions and electrons) and the gas transforms into a mixture of charged particles, i.e. a plasma which is the main constituent of stars and the sun. In the sun, which has an internal temperature of 14 million degrees, the fusion reaction of hydrogen nuclei (proton-proton reaction) is responsible for much of the energy that reaches us in the form of heat and light.
To obtain the fusion reaction, the hydrogen plasma must be confined in a limited space: in the sun this occurs due to the enormous gravitational forces at play. At such high temperatures the problem becomes how to confine the plasma: since there are no containers in nature that can resist in these conditions, magnetic confinement must be used. The particles are forced to follow spiral trajectories around the lines of force of the field, keeping away from the walls of the container: the hot plasma is enclosed in a vacuum chamber, and a suitable configuration of external magnetic fields and/or produced by currents circulating in the Plasma prevents contact with walls.
The European Roadmap towards fusion electricity envisages the construction of the first reactor that feeds electricity into the grid in the second half of the century.
Figure 17. Simplified scheme of a reactor. By U.S.NRC. - http://www.nrc.gov/reading-rm/basic-ref/students/animated-pwr.html, Public Domain, https://commons.wikimedia.org/w/index.php?curid=2320214.
Figure 18. Detailed scheme of a reactor. By Robert Steffens (alias RobbyBer 8 November 2004), SVG: Marlus_Gancher, Antonsusi (talk) using a file from Marlus_Gancher. See File talk:Schema Siedewasserreaktor.svg#License history - Version using font based on File:Schema Siedewasserreaktor.svg, GFDL, https://commons.wikimedia.org/w/index.php?curid=14617356
6. The water-food-energy nexus and climate change
Water, energy and food are essential to human development for reasons that we all know very well. Food and water are essential for life; access to water is necessary for human sanitation. Water and energy are needed for producing and providing food. Energy needs to be produced in a sustainable manner and hydropower is a significant source of energy at the global level. Water and energy are difficult to store and move, while food can be transferred. The above - not exhaustive - examples clarify that there are numerous feedbacks among water, food, energy and humans. To emphasise suck links and retrofits the idea came forward of using a nexus approach, therefore defining the "water-food-energy nexus".
The idea of a nexus approach to water, energy and food security (WEF nexus) was first presented at the Conference "The Water, Energy and Food Security Nexus - Solutions for the Green Economy", held in Bonn during 16 – 18 November 2011 (see here the background paper of the conference by H. Hoff). A Nexus approach focuses on the complex and dynamic interrelationships between water, energy and food by looking at the impacts that a decision in one sector has on the full plurality of involved sectors. The nexus approach anticipates potential trade-offs and synergies, therefore allowing one to prioritise response options that are viable across different sectors. A nexus approach integrates management and governance across sectors and scales. A nexus approach can support the transition to different forms of economies which aims, among other things, at resource use efficiency and greater policy coherence. Given the increasing interconnectedness across sectors and in space and time, a reduction of negative economic, social and environmental externalities can increase overall resource use efficiency, provide additional benefits and secure the human rights to water and food. The nexus approach focuses on environment, economy and stakeholder dialogue (see Figure 18).
Figure 18. The FAO approach to the water-food-energy nexus (from The Water-Energy-Food Nexus - A new approach in support of food security and sustainable agriculture, Food and Agriculture Organization of the United Nations Rome, 2014, retrieved from http://www.fao.org/3/a-bl496e.pdf).
For the reasons outlined above, the WEF nexus is extremely relevant to sustainable development. We present here below some figures related to sustainability of the uses of water, energy and food.
6.1. Sustainability of energy production and use
Energy development is the field of activities focused on obtaining energy from natural resources. These activities include production of conventional, alternative and renewable sources of energy, and the recovery and reuse of energy that would otherwise be wasted.
Download the powerpoint presentation of this lecture
Download a powerpoint presentation on nuclear energy
Last modified on April 29th, 2024
- 821 views